Dirty crumbling
snowballs
By Dr. Nalin Samarasinha
The close approach to Earth of a fragmented comet
called Schwassmann-Wachmann 3 (hereafter called comet SW3), during
mid-May generated worldwide excitement among astronomers and the
general public. Such celestial events kindle the natural human curiosity
to understand the world around us.
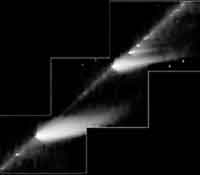 |
This false-colour infrared picture from the Spitzer Space
Telescope, taken in early-May, shows multiple fragments of comet
SW3 acting as individual comets. The bright line represents
dusty material along the orbital path, and is normally seen
in infrared light (image courtesy of NASA/JPL-Caltech) |
Comet SW3, which has an orbit that nearly intersects
with Earth’s orbit, came to within about 10 million km from
us (a relatively close distance in cosmic terms). The comet was
broken into multiple pieces about ten years back. The close approach
to Earth allowed astronomers to have a detailed look at this fragmented
object. Indeed it turned out to be a great show. During the coming
months, astronomers will analyse the observations of this comet
to unravel many mysteries. In this context, we will review the current
understanding of comets, and the relevance of studying these celestial
wanderers to the human race.
What is a comet?
The most important part of a comet is the solid object in its core,
known as the nucleus, which contains dirt and ices. Metaphorically
speaking, this is both the brain and the heart of a comet. As the
nucleus comes close to the sun in its orbit, the volatile ices in
the nucleus sublimate. Sublimation is the physical process, under
which ice transforms into gas, without becoming a liquid; the ‘zero
pressure’ environment in space causes all ice species (including
water ice), to undergo this transition, as the temperature increases.
The sublimating gases drag dust particles from the nucleus into
the ‘atmosphere’ of the comet, which is more commonly
known as the coma. Some gas molecules in the coma get ionized, and
are hauled away in the direction opposite to the sun by the Solar
Wind. This process creates the ion tail, which is also known as
the plasma tail. Meanwhile, dust particles in the coma are pushed
away by the sunlight (i.e., by the solar radiation pressure), generating
the dust tail. As a comet comes close to the sun, it loses a small
fraction of its mass, but generates a beautiful coma and the characteristic
tails.
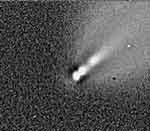 |
This is a computer-enhanced image of the second
brightest fragment taken by the author and his colleagues using
a 4-metre telescope. The two bright clumps along the tail indicate
that this fragment is undergoing further fragmentation. This
confirms that comets are indeed fragile |
An average cometary nucleus is only a few km across
(nominally the size of Colombo city), whereas the coma and the tails
can extend up to a few hundred thousand km and few million km, respectively.
These large numbers are best understood by comparison to familiar
dimensions. If we scale down the nucleus to the size of a potato,
then the size of the coma is comparable to the city of Colombo,
and the tails could span the length of Sri Lanka. Therefore, the
coma of a comet is much larger than the nucleus. In contrast, the
Earth’s atmosphere is a thin shell in relation to the size
of Earth. Unlike Earth’s atmosphere, the dusty gas environment
of the coma (or the tails) is a rarefied medium – almost close
to a vacuum. A spacecraft could fly through the coma or the tails
of a comet, without experiencing any significant slowing. The dust
particles in the coma or in the tail show their presence via reflected
sunlight, whereas gas molecules emit light via fluorescence (the
same physical mechanism through which a fluorescent light bulb generates
light). Thus, the dust tail appears yellow. The plasma tail is blue,
because of the strong fluorescence emission of blue light by carbon
monoxide ions (CO+). In contrast, the coma of a comet may appear
yellow (if the comet is dust rich), or bluish green (if it is gas
rich). The coma of a gassy comet appears bluish green, because the
dominant emission is by CN and C2 molecules in the blue and green
part of the spectrum. Although they may determine the colour, CN
and C2 are not the most abundant species.
So, what are the most abundant species of a comet?
As noted earlier, a cometary nucleus consists of dust and ices.
The most abundant ice is water (H2O) ice. In addition, we have ices
corresponding to many other species. In 1950, American astronomer
Fred Whipple suggested that a comet (or more precisely the comet
nucleus) is a conglomeration of ices and dirt. While this ‘dirty
snowball model’ of the nucleus withstood subsequent scientific
tests, the first direct confirmation was in 1986, when spacecraft
obtained clear images of the nucleus of comet Halley. Due to the
scientific advances catalysed by his work, many consider Fred Whipple,
who died in 2004 at the age of 97, to be the father of modern cometary
science.
How and where were comets formed?
When the Solar System was formed 4.6 billion years ago from a collapsing
cloud of primordial gas and dust, the material accreted, to form
the sun, planets and the moons. The smaller bodies, which did not
grow large enough to become planets or large moons, ended up as
asteroids and cometary nuclei. Asteroids were formed in the warmer
inner part of the Solar System interior to the orbit of the planet
Jupiter. The cometary nuclei with ices, formed in the cooler environment,
approximately beyond the orbit of Jupiter. The early Solar System
was a hazardous environment with a high concentration of asteroids
and comets. For example, Earth and the other planets were bombarded
regularly and at an intense rate by these objects. While such an
environment may not be conducive to unhindered evolution of life,
the impacting comets and asteroids did provide Earth with many complex
organic molecules, which facilitated the eventual biochemical evolutionary
processes.
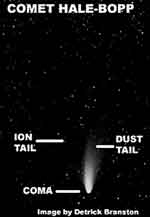 |
This image of comet Hale-Bopp, taken when
it was visible to the unaided eye in 1997, shows the basic components
of a comet. The nucleus, too small to be seen, is embedded in
the middle of the coma |
The largest planet, Jupiter and to a lesser extent
Saturn, Uranus and Neptune were responsible for ‘clearing’
most of the comets and asteroids from the Solar System. The gravitational
forces of these giant planets scattered the comet nuclei that formed
in the giant planet region. Many of the comet nuclei, which were
scattered by giant planets escaped our Solar System altogether.
However, a significant number remained in the outer edges of the
Solar System – a region we call the Oort Cloud, after Dutch
astronomer Jan Oort, who first postulated such a reservoir of comets
nearly fifty six years ago.
At about the same time, astronomers (Ex: the Irish astronomer Kenneth
Edgeworth and Dutch American astronomer Gerald Kuiper) wondered
why the Solar System abruptly ends around 30 Astronomical Units
(an Astronomical Unit, denoted by AU, is the average distance between
the sun and the Earth and equals to 150 million km).
They suggested the possibility of a belt of comets
beyond Neptune in our Solar System. However, it was only in 1992
that the first new object belonging to the Kuiper Belt (also known
as the Edgeworth-Kuiper Belt) was discovered. After the discovery
of this object, 1992 QB1, by David Jewitt and Jane Luu of University
of Hawaii, we now know of nearly 1,000 such objects called Trans-Neptunian
Objects (TNOs). With a sufficient number of TNOs discovered, we
are beginning to see the structure of the Kuiper Belt. In addition,
we now have clear evidence based on the orbits of these TNOs, that
planet Pluto is definitely a member of the Kuiper Belt. This leads
to the interesting question: should Pluto be called a planet, and
if that is the case, should the recently discovered TNO, 2003 UB313
(nicknamed Xena), which by all means is larger than Pluto, be called
a planet too? Currently the International Astronomical Union is
debating the definition of a ‘planet’.
The Kuiper Belt and the Oort Cloud are the two
reservoirs of comets. Due to gravitational perturbations, orbits
of comets in these reservoirs get changed over many millions of
years. This causes some comets to end up with such orbits that make
those comets visit the inner Solar System and Earth’s neighbourhood.
Space missions to comets
During the last 20 years, there were a number of space missions
to comets. They provided a wealth of information on these cold remnants
from the past. However, these missions were flybys, limiting close
observations to a short time interval. The first spacecraft to rendezvous
a comet, and land on it, will be the European Space Agency’s
Rosetta mission to comet Churyumov-Grasimenko.
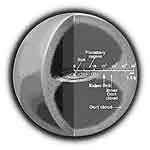 |
This diagram shows the locations and basic
character of the Kuiper Belt and the Oort Cloud. Note that the
distance from the sun is expressed in a logarithmic scale. On
a more familiar linear distance scale, the Oort Cloud would
appear much further away compared to the Kuiper Belt than shown
in the diagram |
Thus far, there have been a number of missions
to comets and four cometary nuclei (Halley, Borrelly, Wild 2, and
Tempel 1) were imaged. These images had sufficient resolution to
identify basic surface features, and for comparisons between individual
nuclei. Interestingly, most nuclei were found to be highly irregular
and elongated.
The last flyby mission Deep Impact, in addition
to taking images and spectra of both the nucleus and the coma, carried
out a novel experiment to explore the subsurface material of the
nucleus by striking it with a copper impactor and excavating a crater.
This general concept is not new. For example, in 2001: A Space Odyssey,
Sir Arthur C. Clarke describes a similar experiment on an asteroid.
Why do we want to explore the subsurface material?
Though comets are the most pristine objects leftover from the formation
of the Solar System, the surface layers, especially, are highly
evolved due to heat from the sun. Therefore, in order to learn about
the more pristine material, one has to look sufficiently interior
to the surface.
The cometary impact by the Deep Impact spacecraft
on July 4, 2005 provided direct evidence that at least the surface
layers of the nucleus consist of more dust than we anticipated.
It also confirmed what many cometary scientists were suspecting
about the density of cometary nuclei based on theoretical work –
the comet nucleus is lighter than water ice. The derived density
was only half that of water. Considering that the nucleus is made
up of dust and ice, what does this mean? Does nucleus contain large
porous structures?
The Rosetta mission will hopefully provide an answer
in 2014, when its radar performs a ‘cat scan’ of comet
Churyumov-Gerasimenko. Knowledge of the cometary structure is relevant,
not only to understand the environment of the Solar System during
its formation phase, but also to devise effective mitigation strategies
for killer comets (or asteroids) that could inflict devastation
in the case of a collision with Earth.
About the writer |
Dr. Nalin Samarasinha is the first native-born Sri Lankan
to have an asteroid named after him, when the International
Astronomical Union renamed Asteroid (12871) 1998 ML37 as (12871)
Samarasinha. The only other Lankans with asteroids named after
them are the Late Prof. Ganesh Chanmugam and Sir Arthur C. Clarke.
After
obtaining an Honours degree in Physics at the University of
Colombo, Dr. Samarasinha did his doctoral research at the University
of Maryland, College Park (USA).
Beginning with his demonstration of the excited rotational state
of 1P/Halley, Dr. Samarasinha, who is a researcher at the National
Optical Astronomy Observatories, Tucson, has carried out many
studies of the dynamical evolution of cometary nuclei. |
|